Click Here To Get Presentation
Abstract
Diabetes has two common forms, diabetes mellitus (type II) and diabetes insipidus (type I). Blood glucose levels above normal marks the onset of diabetes [1]. Over 150 million patients worldwide are affected by type II diabetes (T2D) which is progressive in nature [2]. It is characterized by chronic persistent hyperglycemia and dyslipidemia, which if left untreated leads to diabetic neuropathy and retinopathy in addition to cardiovascular complications [2]. Various agents including thiazolidinediones, biguanides, α-glucosidase inhibitors, sufonylureas and meglitinides seem to not benefit the microvascular as well as the macrovascular risk associated with diabetes [3]. Majority of T2D patients require a combination of drugs as no single marketed drug is able to achieve control over blood glucose levels [3]. Thus there is still a medical need for novel oral agents with more robust glucose lowering efficacy.
Glucokinase (GK) is mostly expressed in the pancreatic β-cells [4] and the liver hepatocytes. Liver and pancreatic GK differ only in the amino acid sequence at the extreme amino terminus and, thus, these tissue isoforms appear enzymatically and kinetically identical [5]. GK is a 465 amino acid residue, 50-kD monomeric enzyme that catalyses the ATP-dependent phosphorylation of glucose to glucose 6-phosphate as the first and rate-limiting step in the glycolytic pathway [6]. GK is often referred to as the ‘glucose sensor’ as it directly relates the rate of β-cell glucose-dependent insulin secretion and the rate of hepatocyte glucose metabolism to the ambient blood glucose level [5]. The role of Glucokinase as a glucose sensor is due to its allosteric properties. It demonstrates sigmoidal glucose dependence instead of non-Michaelis-Menten kinetics and displays no inhibition by glucose 6-phosphate, the product of the reaction. These particular features make GK an ideal sensor of physiological changes in blood glucose levels [7].
In humans, more than 150 naturally occurring mutations in GK have been reported [7]. It has been shown that mutations in a single GK gene allele that impairs enzyme function and causes type 2 maturity onset diabetes of young (MODY2), whereas inactivating mutations in both GK alleles cause permanent neonatal diabetes [8]. In addition, GK mutations that increase enzyme activity cause hyperinsulinemic hypoglycemia [9].
Several glucokinase activators (GKAs) were identified recently had effect similar to the active mutations. A conformational transition between the closed (active) and superopen (inactive) states determined through x-ray crystallography may be linked with the allosteric characteristics of GK [7].
The recognition that GK plays a major role in the control of blood glucose led to drug discovery programs designed to identify small molecules that target GK as potential blood-glucose-lowering drugs for T2D [10].
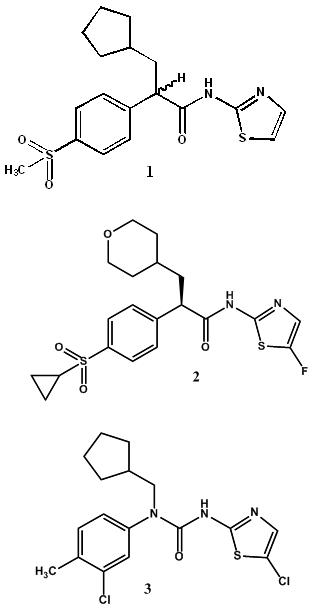
Several glucokinase activators (GKAs) are presently at various stages of clinical development. Hillard et al. [11] screened a library of 120,000 structurally diverse compounds of which one increased enzymatic activity of glucokinase ; its chemical optimization led to the synthesis of compound 1. The R-enantiomer was a potent activator with an EC50 of 0.69 μM whereas the S enantiomer was inactive. Fyfe et al. [12] reported a related series of activators (e.g. 2; EC50 = 0.13 μM). Compound 2 advanced to Phase 2 clinical studies and was found to effectively lower fasting and postprandial glucose in Type II Diabetes (T2D) patients [13]. To avoid the chirality of 2 at the α-carbon Fyfe et al. [14] replaced it with an achiral nitrogen atom and thus discovered urea containing GKAs compound 3 (EC50 = 0.4 μM). A variety of other structurally diverse GKAs have been identified, including aryl amides [15].
Although glucokinase activators (GKAs) have been proposed as a promising therapy for the potential treatment of diabetes, it has yet to be confirmed that the same small molecule activators modulate glucokinase activity simultaneously in liver, pancreas and brain. Because of their dual pancreatic and hepatic actions it is anticipated, however, that GKAs are capable of eliciting their desired glucose-lowering effects when used as monotherapy [16]. Regardless of their usefulness as effective drugs, GKAs have a great potential as molecular tools for studying the function and physiology of insulin secretory cells such as β-cells and other glucose-sensitive cells.
In conclusion, the fact that GKAs affect glucose homeostasis in the liver and pancreatic β-cells makes them attractive targets for potential treatment of T2D. Cocrystal structures of GK with its activators have radically improved the knowledge of GK structure and function thus helping the medicinal chemist to develop and design novel and promising activators. Several small molecule GKAs have been discovered that have shown promising glucose-lowering effects both in vitro and in vivo. The GKAs have the potential to become a new addition to the already established antidiabetic drugs to fight T2D [16].
References
- Mohler, M.L.; He, Y.; Wu, Z.; Hwang, D.J.; Miller, D.D. Recent and emerging anti-diabetes targets. Med. Res. Rev. 2009, 29, 125-195.
- Sarabu, R.; Berthel, S.J.; Kester, R.F.; Tilley, J.W. Glucokinase activators as new type 2 diabetes therapeutic agents. Expert Opin. Ther. Patents 2008, 18(7), 759-768.
- Iino, T.; Tsukahara, D.; Kamata, K.; Sasaki, K.; Ohyama, S.; Hosaka, H.; Hasegawa, T.; Chiba, M.; Nagata, Y.; Eiki, J.; Nishimura, T. Discovery of potent and orally active 3-alkoxy-5-phenoxy-N-thiazolyl benzamides as novel allosteric glucokinase activators. Bioorg. Med. Chem. 2009, 17, 2733-2743.
- Toyoda, Y.; Yoshie, S.; Shironoguchi, H.; Miwa, I. Glucokinase is concentrated in insulin-secretory granules of pancreatic B-cells. Histochem. Cell Biol. 1999, 112, 35-40.
- Coghlan, M.; Leighton, B. Glucokinase activators in diabetes management. Expert Opin. Investig. Drugs 2008, 17(2), 145-167.
- Weber, I.T., Mahalingam, B.; Cuesta-Munoz, A.; Davis, E.A.; Matschinsky, F.M.; Harrison, R.W. Structural model of human glucokinase in complex with glucose and ATP. Diabetes 1999, 48, 1698-1705.
- Shen, X.; Jiang, H.; Zhang, J.; li, C.; Chen, K.; Zhu, W.; Conformational transition pathway in the allosteric process of human glucokinase. PNAS (US) 2006, 103(36), 13368-13373.
- Efanov, A.M.; Barrett, D.G.; Brenner, M.B.; Briggs, S.L.; Delaunois, A.; Durbin, J.D.; Giese, U.; Gua, H.; Radloff, M.; Gil, G.; Sewing, S.; Wang, Y.; Weichert, A.; Zaliani, A.; Gromada, J. A Novel Glucokinase Activator Modulates Pancreatic Islet and Hepatocytes Functions. Endocrinology 2005, 146, 3696-3701.
- Kamata, K.; Mitsuya, M.; Nishimura, T.; Eiki, J.; Nagata, Y. Structural basis for allosteric regulation of the monomeric allosteric enzyme human glucokinase. Structure 2004, 12, 429-438.
- Agius, L. Glucokinase and molecular aspects of liver glycogen metabolism. Biochem. J. 2008, 414, 1-18.
- Hillard, D.W.; Grimsby, J.; Sarabu, R.; Corbett, W.L.; Haynes, N.; Bizzaro, F.T.; Coffey, J.W.; Guertin, K.R.; Kester, R.F.; Mahaney, P.E.; Marcus, L.; Qi, L.; Spence, C.L.; Tengi, J.; Magnuson, M.A.; Chu, C.; Dvoroznaik, M.T.; Matschinsky, F.M.; Grippo, J.F. Allosteric activators of glucokinase: potential role in diabetes therapy. Science 2003, 301, 370-373.
- Fyfe, M.C.T.; White, J.R.; Taylor, A.; Chatfield, T.R.; Wargent, E.; Printz, R.L.; Sulpice, T.; McCormack, J.G.; Procter, M.J.; Reynet, C.; Widdowson, P.S.; Wong-Kai-In, P. Glucokinase activator PSN-GK1 displays enhanced antihyperglycemic and insulinotropic actions. Diabetologia 2007, 50, 1277-1287.
- Pfefferkorn, J.A.; Lou, J.; Minich, M.L.; Filipski, K.J.; He, M.; Zhou, R.; Ahmed, S.; Benbow, J.; Perez, A.; Tu, M.; Litchfield, J.; Sharma, R.; Metzler, K.; Bourbonais, F.; Huang, C.; Beebe, D.A.; Oates, P.J. Pyridones as glucokinase activators: Identification of a unique metabolic liability of the 4-sulfonyl-2-pyridone heterocycles. Bioorg. Med. Chem. Lett. 2009, 19, 3247-3252.
- Fyfe, M.C.T.; Castelhano, A.L.; Dong, H.; Gardner, L.S.; Kamikozawa, Y.; Kurabayashi, S.; Nawano, M.; Ohashi, R.; Procter, M.J.; Qiu, L.; Rasamison, C.M.; Schofield, K.L.; Shah, V.K.; Ueta, K.; Williams, G.M.; Witter, D.; Yasuda, K. Glucokinase-activating ureas. Bioorg. Med. Chem. Lett. 2005, 15, 1501-1504.
- Iino, T.; Hashimoto, N.; Sasaki, K.; Ohyama, S.; Yoshimoto, R.; Hosaka, H.; Hasegawa, T.; Chiba, M.; Nagata, Y.; Eiki, J.; Nishimura, T. Structure-activity relationship of 3,5-disubstituted benzamides as glucokinase activators with potent in vivo efficacy. Bioorg. Med. Chem. 2009, 17, 3800-3809.
- Pal, M. Recent advances in glucokinase activators for the treatment of type 2 diabetes. Drug Discovery Today 2009, 14(15-16), 784-792.