Click Here To Get Presentation
By: Preetpal S. Sidhu
Copper catalyzed alkyne-azide cycloaddition (CuAAC) is one of the most reliable click reaction that has enabled the practical and efficient preparation of 1,4-disubstituted 1,2,3 triazoles from a wide range of substituted alkynes and azides which cannot be typically attained by the traditional Huisgen thermal cycloaddition reaction[1]. The reaction defined as “click reaction” requires benign reaction conditions, simple work up and purification procedure and can still rapidly creates molecular diversity through the use of reactive modular building blocks [2].
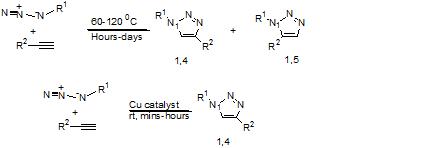
Thermal 1, 3 cycloaddition a reaction give a mixture of region-isomers (Scheme I) and requires the elevated temperatures and long reaction times [3]. The copper-catalyzed 1, 3 cycloaddition reaction preferably gives 1, 4 regioisomer. Copper catalysis increases the rate of reaction up to 107 fold at room temperature [4]. Copper is present in the +1 oxidation state. The reaction can tolerate a wide variety of functional groups in both reactants and works well in many solvents [5]. Interestinly, the reaction perform best in aqueous system, succeeds over a fairly temperature range and is remarkably insensitive to pH [6].
The sources of copper [I] catalyst can be copper [I] salts such as CuI etc [5]. Cu (I) can be produced in-situ by reduction of copper [II] salts [6] or can also be obtained through comproportionation of Cu [0] and Cu [II] salts [6]. It can also be obtained from oxidation of Cu [0] salt [7]. Some time ligand-assisted Cu [I]-catalysis is also used in which the ligand shields the Cu [I] ion from the interactions that can lead to degradation. This significantly reduces the amount of catalyst necessary for the reaction. Amine triazole was the first reported as the most efficient ligand for cycloaddition catalysis [8]. Ligands also add enantioselectivity to ‘click chemistry’. Ligand accelerated cycloaddition appears to facilitate bioconjugation studies.
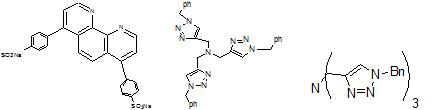
Although CuAAC does not require heating, microwave chemistry can dramatically reduces the reaction time from twelve hours to less than an hour. Usually there is no additional gain in yield but it can reduce some undesirable side reactions [9]. The reaction of sulfonyl azides with terminal alkynes is an interesting exception. Depending on the reaction condition, it yields different products. N-sulfonyl azides are converted to N-sulfonyl amidines in presence of amines [10]. Similarly, in presence of water, N-acylsulfonamide is major products [11]. N-sulfonyl triazole can be obtained in good yield when reaction is performed in chloroform in presence of 2, 6-lutidine [12].
Problem: A by-product is formed due to alkynes homocoupling. This coupling is also catalyzed by Cu [13]. Also, in case of highly electron deficient azide binding to Cu-acetylene complex is less favorable and hence side product formation occurs [14]. The yield reduces for polyalkene substrates that have the flexibility to coordinatively saturate copper [I] [13].
Applications: ‘Click chemistry’ has been employed in synthesis of small molecule screening libraries to derive inhibitors of fucosyl transferases [15] and HIV-I protease inhibitor [16] as well as to design size specific ligands for mRNA hairpin loops [17]. The other various uses are modification and biological profiling of natural products. Many bioactive natural products have narrow therapeutic window which limits their therapeutic potential. Therefore modification of natural products is viable approach to improve their therapeutic index. In, reality, most chemical transformations are not compatible with a range of functional groups present in the parent compound. The high selectivity and fidelity of the CuAAC make it a good reaction for the last-step derivatization of complex bioactive molecules [18].
Bioconjugation is incorporation of amino acids containing small bioorthogonal groups into the proteome by metabolically or through site directed mutagenesis, which allow the tracking of proteome dynamics in response to external stimuli. CuAAC is used to selectively label the unnatural amino acids which are incorporated during synthesis of protein [19]. CuAAC is also used in synthesis of functional dendrimers like peptidodendrimers, unprotected glycodendrimers, redox-active dendrimers etc for creating bioactive nanomaterial or sensor application [20].
References:
1) E. J.Yoo, M.A. Ahlquist, S.H. Kim, I. Bae, V.V. Fokin, K.B. Sharpless, S. Chang, Copper-catalyzed synthesis of N-sulfonyl-1,2,3-triazoles: Controlling selectivity. Angew. Chem. Int.Ed. 2007, 46, 1730.
2). H. C. Kolb , M. G. Finn , K. B. Sharpless ,Click Chemistry: Diverse chemical function from a few good reactions, Angew. Chem. Int.Ed. 2001, 40, 2004
3) K. V. Gothelf , K. A. Jorgensen ,Asymmetric 1,3-Dipolar cycloaddition reaction, Chem. Rev. 1998 , 98 , 863.
4) P. Appukkuttan , W. Dehaen , V. V. Fokin , E. van der Eycken, A Microwave assisted click chemistry synthesis of 1,4 disubstituted 1,2,3 triazole via a copper (I) catalysed three component reaction, Org. Lett. 2004 , 6 , 4223.
5) V. V. Rostovtsev , L. G. Green , V. V. Fokin , K. B. Sharpless, A Stepwise Huisgen cycloadditionprocess: copper (I) catalysed regioselective “ Ligation” of azide and terminal alkyne, Angew. Chem. Int. Ed. 2002 , 41 , 2596 -2599 .
6)C. W. Tornøe , C. Christensen , M. Meldal, Peptidotriazole on solid phase:1,2,3-Triazole by regiospecific copper (I) catalysed cycloaddition of alkyne and azide, J. Org. Chem. 2002 , 67 , 3057.
7)F. Himo , T. Lovell , R. Hilgraf , V. V. Rostovtsev , L. Noodleman , K. B. Sharpless , V. V. Fokin, Copper (I) catalysed synthesis of azoles.DFT study predicts unprecedented reactivity and intermediates, J. Am. Chem. Soc.2005 , 127 , 210.
8)T. R. Chan , R. Hilgraf , K. B. Sharpless , V. V. Fokin, Polytraizoles as copper(I) stabilizing ligands in catalysis, Org.Lett. 2004 , 6 , 2853.
9) F.P. Balderas, M.O. Munoz, J.M. Sanfrutos, Multivalent Neogylcoconjugates by Regiospecific Cycloaddition of Alkyne and Azide by using Organic soluble cupper catalysts, org. lett, 2003, 5, 1951.
10)I. Bae, H. Han, S. Chang, High Efficient one-pot synthesis of N-sulfonylamidines by cu-catalysed three–component coupling of sulfonyl azide, alkyne, and amine, J. Am. Chem. Soc. 2005, 127, 2038.
11)S.H. Cho, E.J. Yoo, I. Bae, S. Chang, Copper-Catalyst Hydrative Amide Synthesis with Terminal Alkyne, Sulfonyl Azide, and Water, J. Am. Chem. Soc. 2005, 127, 16046.
12)E.J. Yoo, M. Ahlquist, S.H. Kim, I. Bae, V.V. Fokin, K.B. Sharpless, Copper-Catalyst Synthesis of N-Sulfonyl-1,2,3-triazoles: Controlling Selectivity, Angew. Chem. Int. Ed. 2007, 46, 1730.
13) E.-H. Ryu , Y. Zhao, Efficient synthesis of water soluble calixarenes using click chemistry, Org. Lett. 2005, 7 , 1035.
14)Y.-M. Wu , J. Deng , X. Fang , Q.-Y. Chen,Regioselective synthesis of fluoroalkylated 1,2,3-triazole by huisgen cycloaddition, J. Fluorine Chem. 2004 , 125 , 1415.
15)Sears, P; Wong, C.H, Enzyme action in glycoprotein processing. Mol. Life Sci 1998, 54, 223.
16)Brik, A; Muldoon, J; Lin, Y.C; Elder,J.H: Olson,A.J; Fokin, V.V; Sharpless,K.B, Rapid diversity oriented synthesis in microtiter plates for insitu screening of HIV protease inhibitor, ChemBioChem, 2003, 4, 1246
17)Thomas, J. R; Liu, X; Hergenrother, P. J, Size specific ligands for RNA hairpin loops, J. Am. Chem. Soc. 2005, 127, 12434.
18) Lin, H; Walsh, C. T, A Chemoenzymatic approach to gylcopeptide antibiotics, J. Am. Chem. Soc. 2004, 126, 13998.
19)Kiick, K. L; Tirrell, D. A, Incorporation of azide into recombinant proteins for chemoselective modification by the staudinger ligation, Tetrahedron 2000, 56, 9487.
20)P. Wu, V.V. Fokin, Catalytic Aide-alkyne cycloaddition: Reactivity and Application, Aldrichimica Acta,2007, 40, 7.