Click Here To Get Presentation
Abstract
Amines are a valuable class of compounds. Amines as end products and as intermediates, like enamines or imines, are used in industrial-relevant processes [1]. There are various methods reported in the literature for the synthesis of amines like a) nucleophilic substitution of alkyl halide with metal cyanide or metal azides followed by catalytic reduction, b) amination using alkyl halides and substituted amines in the presence of catalyst, c) reductive amination of carbonyl compounds, and d) reduction of nitroalkanes [2]. These are reactions which have either stoichiometric salt formation or nitrogen release. Thus the atom efficiency is between 26-60% and the reaction might not be environmentally friendly. In contrast a) hydrocyanation of unsaturated hydrocarbons by hydrogen cyanide in the presence of a catalyst followed by catalytic reduction, b) amination using alcohols and substituted amines in presence of catalyst and c) hydroaminomethylation of unsaturated hydrocarbons are more environmentally friendly and proceed in a relatively higher atom efficiency of 70-100%. All the above methods have either a problem with toxic waste produced or multiple steps required to carry out amination which prompted scientists to look for more efficient ways for synthesis of amines [2].
One of the more efficient approaches to the synthesis of amines is hydroamination reactions. It is an economical, 100% atom efficient process for the synthesis of substituted amines in a single step. It involves addition of primary or secondary amines or ammonia to a carbon-carbon multiple bond (Figure 1) [3]. The hydroamination reaction is carried out using readily available alkenes/alkynes. The [2 + 2] cycloaddition of N-H across the C-C bond is orbitally forbidden under thermal conditions because of the high energy difference between π(C=C) and σ(N-H) bonds energies. Amines can undergo a direct nucleophilic addition to the C-C triple bond if the π system is electron-deficient. This can be achieved by having ketonic (-COR), ester (-COOR), ether (-OR) or nitrile (-CN) as neighboring functional groups. The π bond of alkenes is found to be less reactive towards hydroamination reaction than that of the alkynes [4,5].
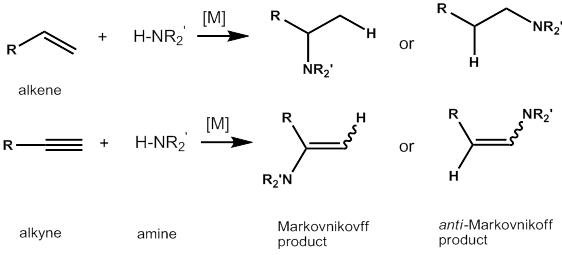
Use of a catalyst [M] can allow a low energy pathway for the reaction to occur in the forward direction and can overcome the difficulties as mentioned above. There are two principal mechanistic pathways to be considered for hydroamination reactions. This involves either activation of the amine or the carbon-carbon multiple bond [6]. Amine activation can be achieved by a) oxidative addition to a transition metal and b) deprotonation of amines using electropositive metal alkalis to give neucleophilic amides. Both methods allow insertion of alkenes to an activated amine. In addition to the activation of amines, C-C multiple bond activation through co-ordination to electrophilic transition metals also allows hydroamination to proceed [2].
Amine and C-C multiple bond activation is achieved using alkali metals like sodium and lithium, early transition metals like zirconium and titanium, lanthanides, and actinides, and late transition metals like ruthenium, rhodium and palladium. In the early-transition metals titanium and zirconium complexes are frequently used. Dimethyl titanocene [CpTi(CH3)2] was found to be widely applicable to primary aryl or alkyl amines and substituted alkynes. Titanium-imido complex is a catalytically active species which allows [2 + 2] cycloaddition of alkynes [7]. Ruthenium, a late-transition metal is most preferred for hydroamination of terminal alkynes. A reaction to synthesize aromatic ketamines was achieved in open air, solvent free, without any inert gas atmosphere. Ruthenium carbonyl [Ru3(CO)12] is used in the presence of small amount of acids or their ammonium salts [8]. Palladium has been the most studied late-transition metal catalyst in hydroamination reactions of various aminoalkynes leading to a variety of nitrogenated heterocycles [9]. Styrene was found to react with various anilines in the presence of acid to give arylethylamines. Alkene activation by co-ordination to the metal followed by amine attack was the mechanism that was proposed [10]. Base-catalyzed hydroamination reactions using alkali-earth metals are more economical because of the lower price of alkali metal derivatives compared to transition metals. Sodium and lithium metals are preferred over the others available. Studies have shown the versatility of these metals to carry out reactions for alkenes and alkynes with either alkyl or aryl amines. The only drawback of these reactions is that reactions are carried out under pressure and at high temperature. Alkali metals activate the amines to the strongly nucleophilic metal amides onto which the alkenes/alkynes add. Further protonation which is believed to follow a concerted pathway by the amine present immediately forms the product [6].
To conclude, hydroamination of alkenes is much more difficult than that of alkynes because of steric reasons and the strength of the π bond which is 70 kJ mol-1 higher than alkynes. To date there has not been one catalyst that can work for most of the C-C multiple- bond compounds. Intramolecular hydroamination reactions are relatively easy to carry out compared to the intermolecular reactions. Intramolecular reactions of aminoalkynes are easier than aminoalkenes, and palladium metal catalyst is most preferred for the former while platinum metal catalyst for the latter. Ruthenium metal catalyst was found to be most preferred for reactions where terminal alkynes were used. Microwave-assisted intermolecular hydroamination reactions using the various above mentioned catalysts have shown to improve the yield and reduce reaction time drastically.
References
- Senn, H.M.; Blchl, P.E.; Togni, A. Toward an Alkene Hydroamination Catalyst: Static and Dynamic ab Initio DFT Studies. J. Am. Chem. Soc. 2000, 122, 40984107.
- Seayad, J.; Tillack, A.; Hartung, C.G.; Beller, M. Base-Catalyzed Hydroamination of Olefins: An Environmentally Friendly Route to Amines. Adv. Synth. Catal. 2002, 344, 795813.
- Nobis, M.; Drieûen-Hölscher, B. Recent Developments in Transition Metal Catalyzed Intermolecular Hydroamination Reactions-A Breakthrough?. Angew. Chem. Int. Ed. 2001, 40, 39833985.
- Muller, T.E.; Hultzsch, K.C.; Yus, M.; Foubelo, F.; Tada, M. Hydroamination: Direct Addition of Amines to Alkenes and Alkynes. Chem. Rev. 2008, 108, 3795-3892.
- Mller, T.E.; Beller, M. Metal-Initiated Amination of Alkenes and Alkynes. Chem. Rev. 1998, 98, 675704.
- Severin, R.; Doye, S. The catalytic hydroamination of alkynes. Chem. Soc. Rev. 2007, 36, 14071420.
- Haak, E.; Bytschkov, I.; Doye, S. Intermolecular Hydroamination of Alkynes Catalyzed by Dimethyltitanocene. Angew. Chem. Int. Ed. 1999, 38, 3389-3391.
- Tokunaga, M.; Eckert, M.; Wakatsuki, Y. Ruthenium-Catalyzed Intermolecular Hydroamination of Terminal Alkynes with Anilines: A Practical Synthesis of Aromatic Ketimines. Angew. Chem. Int. Ed. 1999, 38, 3222-3225.
- Alonso, F.; Beletskaya, I.P.; Yus, M. Transition-Metal-Catalyzed Addition of Heteroatom−Hydrogen Bonds to Alkynes. Chem. Rev. 2004, 104, 3079-3160.
- Kawatsura, M.; Hartwig, J.F. Palladium-Catalyzed Intermolecular Hydroamination of Vinylarenes Using Arylamines. J. Am. Chem. Soc. 2000, 122, 9546-9547.