Introduction:
Proteins are naturally occuring macromolecules made up of repeating units of L-amino acids. There are around 20 different amino acids which are categorized as essential amino acids and non-essential amino acids. Essential amino acids are those which cannot be synthesized by the body and thus have to be provided from the diet whereas non-essential amino acids are synthesized by the body. Essential amino acids include valine, leucine, isoleucine, phenylalanine, tryptophan, threonine, methionine, arginine, lysine and histidine; where as the non-essential amino acids are the remaining from the amino acid pool.
The proteins on degradation break down to individual amino acids which then undergo metabolism and make a significant contribution to the metabolic energy levels (read article: Digestion of dietary proteins in gastro-intestinal tract). Each amino acid type has its own metabolic fate and perform specific functions. Metabolic energy produced varies significantly from herbivores to carnivores to plants. Not only does this metabolic process generate energy, but it also generates key intermediates for the biosynthesis of certain non-essential amino acids, glucose and fat.
Metabolism of Amino acids
Normally the body’s main source of energy is carbohydrates. Amino acids undergo metabolism under three different special situations:
- During the regular synthesis and degradation of proteins, certain amino acids resulting from the breakdown of proteins are not required for the synthesis of new proteins and hence are metabolised.
- When fasting/starving or in situation of patients with uncontrolled diabetes mellitus, deficiency or unavailability of carbohydrates or improper utilization of carbohydrates, cellular proteins are broken down to give energy as an alternative for carbohydrates.
- If a protein rich diet is ingested and the ingested amino acids exceed the body’s requirement for protein synthesis, the excess amino acids are catabolised as they cannot be stored.
In any of the above situations, amino acids get metabolized in the liver by losing the amino group and forming α-keto acids (carbon skeleton of amino acids). These α-keto acids further get metabolised to either three or four carbon units which by gluconeogenesis help produce glucose as a source of energy or can be broken down directly to CO2 and H2O and produce energy. They can also be used to synthesize fat or in the formation of non-essential amino acids which can then be re-utilized by the body. Certain amounts of the amino group released is used for other biosynthetic pathways whereas the surplus is excreted directly (in aquatic animals) or formulated to urea (in many terrestrial vertebrates) or uric acid (in birds and reptiles) depending on the organism which is an excretory end product of protein metabolism. In plants, amino acid metabolism is useful to produce metabolites for other biosynthetic pathways. The figure below summarizes the process of amino acid metabolism.
Discussion of figure above:
The majority of amino acid metabolism occurs in the liver.
- The process of transfer of α-amino group from the amino acid to form keto acid is called as ‘‘transamination” and is catalyzed by the enzyme ”transaminases” or ”aminotransferases”. The α-amino group released is transferred to α-ketoglutarate to give L-glutamate. Glutamate is the only amino acid capable of liberating free NH3 for urea synthesis by oxidative deamination.
- This reaction is a reversible reaction and there is no net deamination (free NH3 produced) as the amino acid is deaminated and α-ketoglutarate gets aminated simultaneously.
- There are different types of aminotransferases each having different specifications for different amino acids, of which aspartate transaminase and alanine transaminase are the most significant ones.
- All aminotransferases require a co-enzyme called pyridoxal phosphate (PLP) which is derived from vitamin B6.
- There are certain amino acids like lysine, proline, hydroxyproline and threonine which do not undergo transamination. Also the process of transamination is not restricted only to α-amino groups, exceptions like δ-amino group of ornithine also take part.
Mechanism of action of transamination/Role of Pyridoxal phosphate (PLP)/Bimolecular Ping Pong Mechanism
- The aldehyde group of pyridoxal phosphate (PLP) is covalently bound by an aldimine linkage (schiff base) to the ε-amino group of lysine residue at the active site of the enzyme aminotransferase.
- The amino group from the amino acid gets temporary bound to pyridoxal phosphate at the active site of the enzyme aminotransferases to form pyridoxamine phosphate. The binding of amino group happens to the aldehyde group of pyridoxal phosphate.
- The amino group from pyridoxamine phosphate is then donated to an α-keto glutarate and PLP is free for the next set of reaction.
- Thus aminotransferases follow bimolecular ping pong mechanism, in which when the first substrate (amino acid) is bound to the enzyme (via PLP) and reacts (pyridoxamine phosphate), the second substrate (α-ketoglutarate) cannot bind to form second product (L-glutamate) till the first product (α-keto acid) leaves. Only once the α-keto acid leaves the active site of the enzyme, can the α-ketoglutarate molecule come into the active site and get converted to form the L-glutamate.
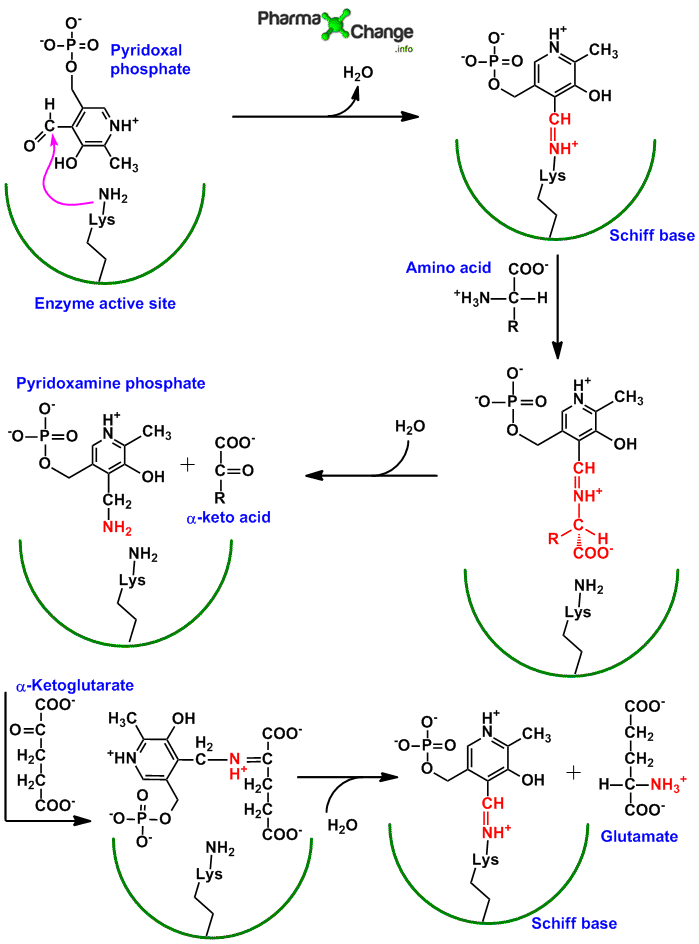
Release of Amino group in Glutamate as NH3/Deamination
Free ammonia is toxic in the body and must be removed by excretion. The amino group is therefore rarely kept free as ammonia within the body and is generally present as a complex with glutamate.
The amino group transferred from the amino acids to glutamate has to be removed in the liver for excretion. The process of removal of the amino group is called as deamination. As the process of transamination and deamination occur simultaneously these reactions can be called as transdeamination. The amino group now has 2 fates as follows:
- Oxidative deamination- It is the removal of amino group as free ammonia coupled with oxidation. In the mitochondria, glutamate dehydrogenase promotes oxidative deamination to convert glutamate to α-ketoglutarate and liberate ammonia through the formation of an intermediate α-iminoglutarate. Glutamate dehyrogenase is the only enzyme which can use either NAD+ or NADP+. The α-ketoglutarate formed is recycled to the Citric acid cycle (Kreb’s cycle). L-amino acid oxidase and D-amino oxidase possessing FMN and FAD are also present to help promote the conversion (oxidation) of glutamate to α-ketoglutarate and ammonia and thus in process the oxygen gets reduced to H2O2.
- Non-oxidative deamination- Certain amino acids (such as serine, threonine, homoserine, cysteine and histidine) without 0xidation get deaminated to liberate ammonia in presence of specific enzymes (dehydratases for -OH containing amino acids, desulfhydrases for sulfur containing amino acids, and histidases for histidine).
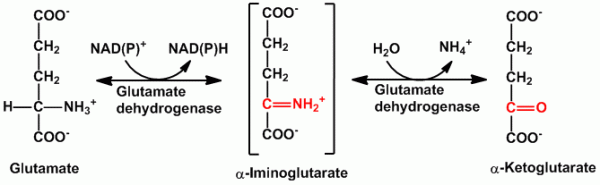
As free ammonia (present as NH4+ ions at physiological pH) which is produced in various extrahepatic tissues is toxic,it is transported to the liver in the form of glutamine or alanine (both are non-toxic). This happens in the extrahepatic cells by the reaction of ammonia with glutamate in presence of enzyme glutamine synthetase to form glutamine. Alanine is formed in the skeletal muscles by the reaction between pyruvate (product of glycolysis) and ammonia in the presence of alanine aminotransferase.
Once transported from the tissue to blood to liver, glutamine is converted back to glutamate and ammonia by the enzyme glutaminase, whereas alanine is converted to glutamate (releases NH4+ by enzyme glutamate deyhdrogenase) and pyruvate (takes part in gluconeogenesis) by giving the amino group to α-ketoglutarate . This free ammonia is converted to urea through the urea cycle and is ready for excretion or the ammonia is used for synthesis of various other compounds in the body.
It is to be noted that therefore, a high protein diet could result in liver damage due to excessive stress on the liver to process the ammonia (in the form of amine) generated from protein metabolism.
Recommended Texts
- David L. Nelson and Michael M. Cox, Lehninger Principles of Biochemistry 6th Edition
- Jeremy M. Berg, John L. Tymockzo and Luber Stryer, Biochemistry 7th Edition
- Reginald H. Garrett, Charles M. Grisham, Biochemistry by Reginald H Garrett 5th Edition
.
- U. Satyanarayana, U. Chakrapani, Biochemistry by U. Satyanarayana. 3rd Edition.